Introduction: Interfacial reactions have become an important subject of research in the past decade. Chemical and biochemical sensing based on such reactions are being widely studied and applied to extend the scope of practical science. Exploring biological interactions on solid support is crucial for a better understanding of biological pathways, designing of biosensors and bioactive surfaces. Such solid supported biological interactions find huge applications in industrial catalysis, drug screening and medical diagnostics.
Immobilization and sensing: Immobilization of biomolecules (proteins, enzymes etc.) on solid support is the first step of such studies and has been carried out by varied means. Several chemoselective methods like native ligation, Staudinger ligation, Diels-Alder and Click reactions and chemoenzymatic methods have been used to graft various proteins on surface. Proteins have also been physically adsorbed on nitrocelluslose membranes for visual sensing. Self-assembled monolayers (SAMs) and metal nanoparticles are vital components of nanoscience which have been extensively used for fixation and labelling of biomolecules respectively. Exploiting specific nature of antigen-antibody binding, antibodies tagged with nanoparticles can be used for sensitive visual detection of antigens, even when present in very low quantities or in complex media.1 This has opened up avenues for the development of assays/detection methods.
Self-assembled monolayers2: SAMs are organic assemblies formed by spontaneous assembly of molecular constituents/surfactants from liquid or gas phases on the surface of solids. These are one molecular layer thick (1-3 nm in thickness). The driving force behind formation of SAMs is the affinity between the head group of the molecule and the substrate surface. When the affinity is strong, it results in formation of a stable film. SAMs can simplify attachment of a target molecule on a solid surface by proper chemical activation of the surface. For this purpose, either a suitably functionalised molecular chain can be synthesized and self-assembled, or post assembly synthetic transformations need to be carried out on a precursor SAM. The latter is simpler and mostly in vogue. Chemical modification of the SAMs and the scope of introduction of virtually any functional group as the tail group of SAMs open up the possibility of tuning surface composition and hence can serve as an invaluable tool for immobilization of different molecules and studying interfacial reactions.
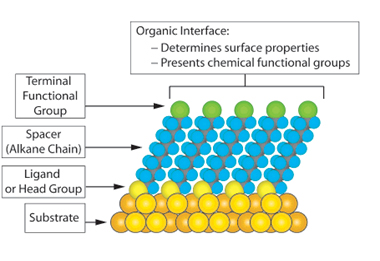
*Image from above mentioned reference (2)
Few examples of bioactive surfaces on SAMs:
- Bacteriolytic surface3: Lysozyme is a bacteriolytic enzyme. In one of our studies, it was covalently immobilised by a mild and convenient chemical reaction on glass and silicon surfaces with almost ̴ 40 % retention of its activity and could be reused without loss of activity. This strategy can be extended and modified to coat surgical devices with bacteriolytic coating which will facilitate sterilising. Such surfaces can also find extensive use in food processing industries.
- Heat shock resistant surface4: This was designed by grafting of a chaperone protein (α-Crystallin) at the terminus of a suitable chemically modified The chaperone activity of immobilized α-Crystallin was measured against aggregation of Aldolase as the target protein. It was found from this study that this heat-shock resistant protein could prevent aggregation of proteins for a wide range of temperatures, showing better activity in immobilised state than in solution. This implies better heat shock resistance at much lower concentrations!! This strategy could make storage and transport of proteins operationally simpler.
- Bio-affinity chips5: Silicon/glass and Au surfaces have been modified with chemically tuneable SAMs that allowed rapid immobilization of protein A on surface, which lead to bio-affinity immobilization of rabbit IgG in an oriented manner. It was shown that by using the protein A chip developed by this protocol, rabbit IgG can be successfully purified from rabbit serum. This chip could also be recycled over 3 cycles. This strategy can be thus used for designing other such chips and can be used to detect target proteins present in low concentration from mixtures of other proteins!! Such systems have come up as a very vital tool in detection and assays.
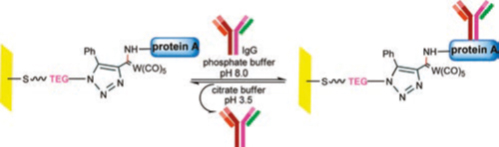
*Image from above mentioned reference (5)
Detection techniques for Covid-19:
- RT-PCR technique: We are all aware of the SARS-CoV-2 and the havoc it has been wrecking. It is a single-stranded RNA virus. As a primary method of detection, the RT-PCR method is being used. For this a nasopharyngeal swab is collected from the patient. An extracted sample from it is treated with an enzyme known as Reverse Transcriptase in presence nucleotides and primers that are complimentary to a specific SARS-CoV-2 target sequence. If viral RNA is present in the sample, the primers bind to them and the enzyme then synthesises a complementary DNA strand. Then the cDNA strand is amplified by PCR technique and can be detected.
- Antibody testing: Another detection strategy is based on antibodies specific to Covid-19. Development of immunity to a pathogen is a time taking process. After a non-specific response via macrophages, neutrophils and dendritic cells to hinder the virus, the body makes an adaptive response by making antibodies (proteins known as immunoglobulins).6 Antibodies collected from blood samples of recovered people can then be used for detection of the virus from test samples.
This is where a bioactive surface could come very handy. To put it simply, if the antigen could be immobilised on a SAM and the antibody tagged to a nanoparticle, their binding could show color to the naked eye and detection may not require sophisticated instruments. It is definitely easier said than done, but science and technology is moving forward in leaps and bounds and most of what is reasonably thought of can be achieved.
References:
- Analytical Methods, 2014, 6, 351–354
- Chemical Reviews 2005, 105, 1103-1169.
- Organic and Biomolecular Chemistry, 2011, 9, 5123–5128
- Bioconjugate Chem. 2014, 25, 888−895
- Bioconjugate Chem. 2011, 22, 1202–1209
- https://www.who.int/news-room/commentaries/detail/immunity-passports-in-the-context-of-covid-19
Visited 1649 times, 1 Visit today